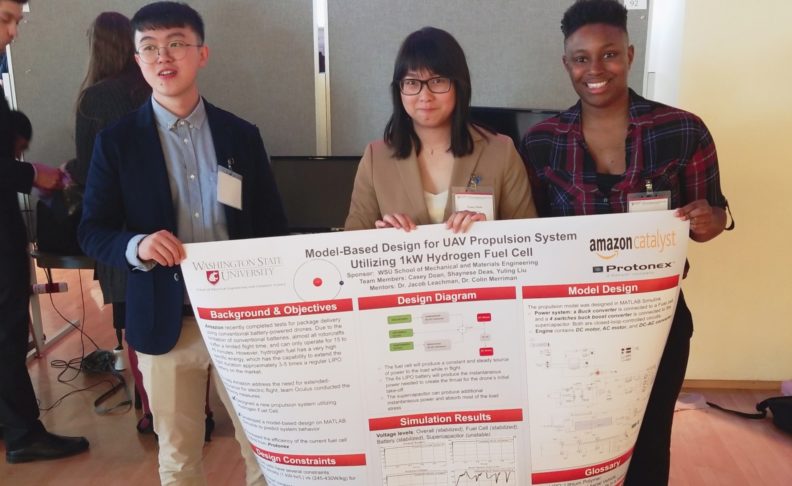
Overview
Setting the >5 kg Electric Rotorcraft Endurance Record via Liquid Hydrogen Fuel Cell Power
Executive Summary
To help Amazon address the need for long-endurance electric flight, a joint team was formed in the fall of 2018 between the Washington State University (WSU) Aerospace Club, Senior Mechanical Engineering Capstone, and Senior Electrical Enginering Capstone programs. The goal of this joint team is to set the >5kg electric rotorcraft endurance record utilizing a liquid hydrogen fuel tank developed by WSU spin-off company Protium Innovations LLC. The project objectives include: 1) Selecting a commercial drone platform to conduct the demonstration, 2) Designing the mechanical interface components to support the fuel tank, fuel cell, and gas manifold from the drone platform, and 3) Designing the electrical control systems to maximize flight efficiency. This document is an initial design report submitted to Amazon and Protonex for review and feedback.
Introduction
The electric rotorcraft delivery industry is at a tipping point. Amazon has recently completed tests of package delivery using conventional LIPO battery powered drones. Hydrogen fuel has a very high specific energy, which can extend the flight duration approximately 3-5 times over regular LIPO battery powered drones on the market. This pairing of hydrogen and drones with Amazon is deliberate as Amazon already maintains liquid hydrogen storage capabilities at most fulfillment centers to tend hydrogen fuel cell forklift fleets. The main goal for this project is to demonstrate a liquid hydrogen fueled drone while setting a flight endurance record for a commercial heavy lift multirotor drone greater than >5 kg in weight.
Background
The WSU Aerospace club began investigating liquid hydrogen fueled drones in 2011 with the Genii drone. The drone, powered by a 1 kW Horizon fuel cell system achieved a total of 18 hours in the air between 9 flight events. The project subsequently attracted the interest of Insitu, a subsidiary of the Boeing Company to develop novel liquid hydrogen fuel tanks for drones.
Figure 1: The Genii hydrogen fueled drone takes off on it’s second flight in March of 2012.
Through grants from the Washington Joint Center for Aerospace Technology and Innovation (JCATI), WSU developed the world’s first 3D printed liquid hydrogen fuel tank. This patented technology utilizes the boil-off vapors from the liquid hydrogen to insulate the vessel while warming the fuel for utilization in a fuel cell. The resulting tank has superior volumetric storage capacity, specific weight, and is robust compared to conventional vacuum jacketed or spray-foam insulated systems. A WSU spin-off company Protium Innovations LLC has developed several of the tanks and is interested in conducting a demonstration of the tank in a rotorcraft drone configuration. With the analysis, design, and manufacture of the tank completed, the challenge for this project is to design the platform for demonstrating the technology. The remainder of this report documents the design selections for the commercial drone platofrom, power system, and mechanical support systems to complete this conversion.
Commercial Platform Selection
The challenge of vehicle platform selection comes down to available powerplant and mission. Most of the Proton Exchange Membrane (PEM) Fuel Cells available for the aerospace market are rated at 1 kW electrical power output. This power output is amenable to a commercial drone optimized for heavy lift in the agriculture and package delivery sectors. These drones typically cost between $12,000-$25,000 and can lift approximately 12-15 kg. The combination of a typical fuel cell and the aforementioned 3D printed liquid fuel tank weighing at most 9 kg. Given the funds available for this project ($13,000) we needed to identify a lower cost platform that still functioned with a comparable lifting capacity to these commercial drones. The DJI S-1000 octocopter baseline configuration starts at $4,000 and has a lifting capacity between 9-11 kg and the 9 kg loading allowing hover at 1500 W. The S-1000 platform is rated for a 6S power supply and matches a Protonex fuel cell. We anticipate being able to “light-weight” the baseline DJI configuration to drop the power requirement at hover to be functioning within the purely “hydrogen” powered capability of the fuel cell. The 300 g capacity of the liquid hydrogen fuel tank would allow approximately 4-5 hours of flight time. We will by second parter components to mount to this DJI S-1000 frame to allow an open-sourced conversion for incorporating the fuel cell. The base DJI system does not allow for generic battery swaps. We can modify this base platform with larger (18”) props and motors to adapt to the power and weight requirements.
Power system
Despite having much higher energy density, the hydrogen fuel cell has lower power density compared to LIPO batteries. As a result, the fuel cell produces less output power and lower current than required to power the motors. The S-1000 E-speed controllers (ESC) and motors will burst to around 80-90A with 60A on average. The Electrical Engineering (EE) team is designing a power flow model in Simulink to determine the suitability of the 1 kW fuel cells for powering the S-1000 platform.
The original H-1000 fuel cell utilized on the Genii drone was tested to determine suitability for this application. After over 10h of hydration utilizing a procedure recommend by Protonex, over 3h was required to reach a peak power generation of 850W. Based on the report from the Genii team back in 2014, during peak performance, the FC had never exceeded 900W. After 2 phone calls with HES, it is highly unlikely the FC will ever reach 900W again. The electrical team suggests moving on to the FCair 1200 FC and stop working on the H-1000.
The EE team is considering adding an ultra-capacitor to the system in order to smooth the power demands on the fuel cell. This decision depends on responses to the following questions:
1) Does Protonex have a report detailing the performance of the FC under similar conditions to those described above? Specifically we need the voltage and current provided by the fuel cell and battery pack combination.
2) The number of cells in the stack.
3) Opportunities for reducing the fuel cell weight.
4) Any addition relevant data the team would need to know.
Tank Manifold, Fuel Cell Bracket, Tank Bracket
The projected weight of an overall product is roughly 9kg (20lbs), making it important to minimize the drone weight to increase payload or hover duration. Keeping the drone well balanced is also going to be one of the objectives. To increase the stability of the drone, a model of the drone frame is being constructed in Solidworks and heavier components will need to be mounted on the lower section of the drone to keep the overall center of gravity as close to the rotor plane as possible.
Another risk is the possibility of the drone losing control mid-flight due to a lack of fuel, loose connection, or outside disturbances, and causing the drone to crash. The hydrogen drone will need to maintain stable flight while outside under at least normal conditions such as a mild wind speed, the ability to maneuver quickly and efficiently as to avoid buildings and other stationary objects, and be able to have a sufficient fuel supply so it can operate for a target time of five hours.
Tank Manifold
To get the hydrogen from the tank to the fuel cell, a manifold is being designed by the mechanical design team for a gaseous hydrogen tank for proof of concept. The tank will hold approximately 2 liters of gaseous hydrogen and will be rated for 1200 PSIG to hold enough gaseous hydrogen to get around 30 minutes of flight time. Pictures of the manifold design are provided. To save weight, there will be no gauge on the tank itself, but there will be one on the filling nozzle. The manifold is designed to comply with the CGA S1.3 Pressure Relief Device Standards and allowed for multiple tanks to be mounted.
Figure 2: Conceptual rendering of the gaseous fuel manifold.
Fuel Cell Mounting Bracket:
The fuel cell bracket is currently designed off of the existing bottom mounting plate for camera gimbals. This plate can not be adjusted as it serves as a ‘motherboard’ transferring power in the S-1000 platform. One design we are considering is 3D printed nylon with a weight of 100 grams. The top of our bracket has two slots that can bolt to the existing slots on the mounting plate. Our current design revolves around certain assumptions on the geometry based on the overall envelope size and a couple of pictures along with the mounting hole size found on the FCair 1200 spec. These adjustments should be easy enough to complete with some additional information from Protonex. FEA under the current assumptions has shown a minimum factor of safety of 23. Additional lightweighting of the bracket will reduced this number.
Tank Bracket:
The tank should be mounted above the drone and the fuel cell below to try and keep the center of gravity close to the level of the propellers. As for the mounting of the tank our current design consists of using clips to attach ¾” thick strips vinyl foam to the top flat of the drone in order to cradle the tank. The tank would then be secured down on these strips using nylon webbing straps and load rated buckles. The straps have a breaking strength of 2,500 lbs and the buckles have a load capacity of 130 lbs.
To have the best result, the ME-team requests the following information:
1) A Computer Aided Drafting (CAD) model of the FC 1200 system to appropriately size the mounting bracket.
2) Location of center of gravity (COG) of the fuel cell system. Is the COG controllable?
Summary and Future Work
The WSU team wants to thank Amazon and Protonex for their interest in this project. We believe we have a design that is ready to implement. The team wants to emphasize their excitement to begin ordering components and constructing the system. The team also wants to express their thanks, in advance, for feedback you have on this project.
The following timeline is anticipated for the remainder of this project:
11/15/18 – Begin ordering system components and sending out mounting brackets for prototype production.
1/1/19 – Begin drone platform assembly and operation with battery power, develop safety plans for benchtop hydrogen testing.
2/15/19 – Begin benchtop testing of fuel cell and drone system, develop safety plans for outdoor tethered flight demonstration.
3/15/19 – Tethered flight demonstrations proceeding to endurance test.
4/15/19 – Submit completed report with results to Amazon Catalyst